3.10 Storage of Water and Nutrients
Waterlogging and Drainage
The degree to which a soil becomes waterlogged
depends on how much water enters the soil and how quickly it leaves,
either by deep percolation, lateral seepage or evapotranspiration.
Consequences of Waterlogging
Waterlogging causes oxygen starvation in the root
zone, which retards root development and may lead to death directly, or to
poor growth and low productivity due to a stunted root system.
Waterlogging also causes nitrogen loss through denitrification and can
affect the availability of other nutrients. Toxins secreted by anaerobic
bacteria, which flourish in waterlogged soils, may also damage plants.
Waterlogged soils are prone to compaction damage by
livestock trampling and vehicles and on sloping ground are susceptible to
erosion due to their poor capacity to absorb water.
Assessing Drainage Conditions
Observation of the soil after heavy rain and during
the late winter to late spring period, provides the best guide to soil
drainage conditions. The most commonly waterlogged soils in South
Australia have sub-soil clay layers of low permeability. Water accumulates
in the coarser material above the clay, forming a perched water table.
These soils are recognisable when dry by dull yellow and grey mottled
colours in the clay and by a dense, bleached layer immediately above it.
Less severe waterlogging can occur in other texture contrast soils (eg
sand over clay) which do not exhibit these colours.
Low lying areas often become waterlogged from run-off
caused by poor infiltration upslope.
Storage of Nutrients
The mineral inorganic component is the largest
fraction of the soil. We have already touched on this component when
texturing the soil, i.e. the sand, silt and clay fractions in the soil.
Of the three mineral components, clay has the
greatest influence on soil characteristics. Clays have a number of special
properties which influence:
-
Total water storage capacity
-
Plant available water
-
Storage of plant nutrients
-
Soil structure and stability
-
Ease of cultivation.
Properties of Clay
Size
Clay particles are extremely small, even the largest
are less than 0.002mm in diameter.
They are so small that, when shaken in water and
allowed to stand, most of the particles do not settle out for a
considerable time, but remain suspended. This is why dam water stays muddy
after it has been stirred up.
Fortunately, it is possible to drop the particles out
of suspension. Certain chemicals, when added to water cause particles to
come together so that their combined weight causes them to sink to the
bottom. For instance, by adding gypsum to muddy dam water we can make the
particles settle out and leave the water clear. This process is called
‘flocculation.’
Many of our soils contain plenty of these
flocculating chemicals, especially calcium compounds, so clay is generally
in a flocculated state. The action of flocculation results in sand and
silt particles being bound together with the clay particles. So, although
clay particles are very small, they are often found naturally bound
together into micro-aggregates less than 0.1mm diameter.
The aim of cultivation practices should be to retain,
not breakdown, aggregates and ensure soil structure is preserved.
Excessive cultivation tends to break the micro-aggregates into individual
particles, blocking pores and resulting in poor infiltration.
In soils with high sodium levels the clay particles
tend to disperse. Therefore. poor structure in the form of huge clods is a
common problem in sodic clays.
These clays can be improved if the sodium on the clay particles is
replaced by calcium. This is what happens when gypsum is used as a soil
amendment to improve soil. A clay can be sodic without being saline, but a
soil containing clay that is subject to saline conditions will probably
become sodic as sodium is forced on to the clay particles at the expense
of other ions. The addition of gypsum can replace the sodium with calcium
on the clay complex.
Shape and Composition
Clay particles are flat crystals made up of many thin
sheets which are tied firmly together by either hydrogen atoms or water
molecules. Mica is a clay mineral with large enough sheets which you can
actually see and peel off.
Figure1:
Vertical section of a clay mineral.
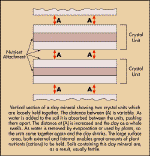
Source: Dept
of Agriculture Bulletin 462, 1960
|
The properties of the clay are determined by the
levels of silicon, aluminium or iron associated with it. For example, high
silicon clays are usually grey, sticky and compact, whereas clays high in
aluminium and iron are usually red, porous and friable.
The type of clay mineral also decides the clay’s
ability to absorb and hold water and to hold plant nutrients.
The Effects of Clay in the Soil
Texture
There is some clay in all soils, eg:
-
Sand may contain 0-9% clay
-
Sandy loam may contain 8-21% clay
-
Loam may contain 10-26% clay
-
Clay loam may contain 21-40% clay
-
Clay soil contains more than 31% clay.
The amount of clay may also vary down the profile.
The sub-soil usually has more, because the fine clay particles have been
washed down by rain over the years.
Water Storage
The sheets of the clay crystal are tied firmly
together, but water is still able to penetrate between them. This means
that soils with a high clay content have a lot more space to store water
than those which are mostly sand. Sand crystals are solid and cannot
absorb water.
However, not all the stored water in clays is readily
available to plants. This is illustrated in Figure 1.
Fertility
Clay particles have a major influence on a soil’s
capacity to store plant nutrients. This comes about because they have a
relatively strong negative charge. Many plant nutrients in the soil
solution have a positive charge. These cations, as they are called, are
attracted to and held by the clay particle. This attraction is strong
enough to prevent nutrients leaching beyond the plant’s reach. The main
cations held in solution are calcium, magnesium, potassium, sodium and
hydrogen.
Plant roots are able to extract and absorb nutrient
cations from the clay surface. This is known as cation
exchange.
Clay minerals can be an important source of essential
nutrients for plants. The more clay in the soil, the more nutrients that
can be stored as cations for future plant use.
The type of clay also has an important bearing on
nutrient storage. For example, the clay minerals associated with the black
earths and red brown earths of the Lower North can store more plant
nutrients (have a higher cation exchange capacity) than the clay minerals
of the ironstone soils of Kangaroo Island and the sandy textured soils of
the South Mount Lofty Ranges.
Ease of Cultivation
Generally, clay soils are hard when dry and sticky
when wet, so they only have a limited range of moisture content suitable
for cultivation. Plasticity varies between clay types. Grey clays are
highly plastic and most difficult to work, but red clays are usually more
friable and easier to work.
Slaking, Swelling and Dispersion
Clay reacts with water in different ways, depending
on the type of clay, the chemical and organic matter associated with it
and the management applied to the soil.
Slaking is the breakdown of aggregates on wetting
into smaller particles. The aggregates shatter because of the pressure
created by the clay swelling and the trapped air expanding inside the
aggregate. Compacted clays lacking organic matter are particularly
susceptible to slaking.
Clay swells when wet because water enters between the
sheets of the crystal. Conversely, when it dries out it shrinks. In some
soils we see the effect of shrinkage as large cracks in the ground.
This property is most marked in our ‘Bay of
Biscay’ soils, which have these properties of shrinkage and swelling to
such a marked degree that they are unsuitable for building, unless special
foundations are used. These soils also cause premature ‘root pruning’
as they stretch roots during dry spells severing some of the plant’s
root system just when it needs it most.
Dispersion is the breakdown of clay micro-aggregates
on wetting, into single particles that are light enough to float in a
milky suspension. A sodic soil in non-saline conditions will form such a
suspension in water and result in crusty or hard at layers in the field.
Figure 2
Exchange of cautions between a root and soil colloids.
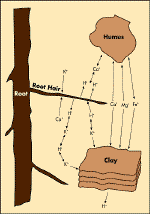
Source: Hi-Fert, Plant Nutrition and Soil Fertility, 1997
|
Organic Matter
Soil organic matter is not just the visible stubble
left behind from the harvest or the dried grass that the animals did not
eat.
It is made up of living roots and soil dwelling
organisms (eg fungi, earthworms, bacteria); decomposed plant, animal and
micro-organism residues; exudates from plant roots and microbes; and
humus.
Organic matter is concentrated in the top 5cm of soil
and contributes to the darker colour of surface soil. It is easily
destroyed by overworking the ground, or lost by allowing water or wind
erosion to occur. Management practices which maintain and build organic
matter are:
-
High yielding broad acre crops and pastures
-
Reduced cultivation
-
Retention of crop residues
-
Green manure crops
-
‘Living’ mulches on bare ground/inter rows
-
Pasture phases within a cropping rotation
-
Avoiding overgrazing of pastures
-
Minimising bare fallows
-
Applications of sewage sludge or animal manure
(watch out for heavy metal contamination)
Many foreign soils have an organic matter content in
the top 10cm (expressed as organic carbon) of >3%. If the organic
matter falls below this level, the fertility will be greatly reduced.
Australian soils, which are geologically very old, are inherently low in
organic matter and very few would approach the 3% organic carbon level.
This is due to the dryness of the climate, high temperatures and lower
fertility status. Over 75% of Australian cropping soils have an organic
carbon level below 1.75%. Practices that help to build organic matter will
increase soil ‘health’.
The Role of Organic Matter
-
Nutrient Store. Nutrients are stored on organic matter and soil
mineral particles by electrical charges. Like clay particles, organic
matter is usually negatively charged and so can attract and loosely hold
positively charged ions (cations).
Organic matter has a very large surface area and,
therefore, many ion exchange sites. The greater the amount of organic
matter in a soil, the more nutrients there will be for plant growth (see Figure
2).
Organic matter holds more than 95% of a soil’s
nitrogen. It can also hold around 90% of the soil’s sulphur and 15 to
85%(normally 30 to 50%) of a soil’s phosphorus. The range for phosphorus
is large because of variations in available amounts of phosphorus held in
the mineral form, bound to iron or calcium. The presence or absence of
organic matter also influences other plant nutrients.
-
Nutrient Supply. Organic matter regulates the supply of plant
nutrients through its ability to form complexes with metal ions. The term
‘complex’ covers a number of chemical interactions between organic
matter and metal ions. Perhaps one of the more important interactions is
called ‘chelation’ because of the way part of an organic molecule
'wraps' itself around a metal ion, holding it in a claw-like chemical
combination.
Organic matter/metal complexes provide a very
effective way of holding the micronutrient elements iron, copper,
manganese and zinc in soil in forms that are readily available to plants,
while protecting them from influences, such as high pH, that would make
them unavailable to plants.
-
Improve soil structure and water holding capacity. Organic
matter improves a soil’s structure by binding soil particles together to
form stable aggregates. The physical and chemical bonds formed protect the
soil aggregates from the destructive forces of rainfall impact, wind
erosion and, to a limited extent, cultivation.
The formation of stable soil aggregates creates gaps
and pores for air and water movement into and through the soil and
provides pathways for root growth.
Organic matter is of greater structural importance in
medium to light textured soils than in clay soils, where the clay itself
helps to bind the soil.
-
Buffer
the soil against rapid change. Another effect of organic matter
in the soil is to act as a buffer to rapid changes in the pH of the soil
solution. This can occur when fertilisers are added to the soil, eg urea.
Organic matter also buffers the soil against rapid
changes in soil temperature that can occur in the lighter soils of inland
areas where day to night temperature variation is large.
Organic carbon percentage is the measurement used to
assess soil organic matter content. Figure 3 illustrates the different percentages, i.e.
different soil types.
Figure 3:
Organic
Carbon (%) (Walkley/Black)
|
Texture
|
Low
|
Moderate
|
High
|
Sand
|
<0.5
|
0.5-1.0
|
>1.0
|
Sandy Loam
|
<0.7
|
0.7-1.4
|
>1.4
|
Loam
|
<0.9
|
0.9-1.8
|
>1.8
|
Clay Loam/Clay
|
<1.2
|
1.2-2.0
|
>2.0
|
Source: Primary Industries and Resources SA
|
Cation Exchange Capacity and Exchangeable Cations
Chemical activity in the soil revolves around the
colloid fraction, comprising the clay minerals and organic matter. Soil
colloids are predominantly negatively charged (anions). Positively charged
ions (cations) can be held by weak electrostatic forces to negatively
charged sites in the soil colloids. The chemical nature of the soil is
determined by the number of negatively charged sites on the surface of the
clay and organic colloids, together with the relative proportions of
different cations on the exchange site.
The most abundant free soil cations are calcium,
potassium, magnesium and sodium. These are important in supplying plant
nutrition. The greater the number of charged sites, measured by the cation
exchange capacity (CEC), the greater the nutrient retention ability of the
soil and the better its capacity to supply cations as nutrients to plant
roots.
Cation exchange capacities of more than 15 indicate
high soil fertility; values of less than five indicate low fertility.
The proportions of the various cations on the
exchange complex are also important. Excessive exchangeable sodium, as
well as causing structural problems (sodicity), affects plant growth due
to the low tolerance of many species to high sodium. High magnesium to
calcium ratios are also undesirable.
Between 1-5% of soil particles are positively
charged. Negative ions can become attached to these, but generally stay in
the soil solution and are subject to being leached from the plant root
zone. Nitrates (NO3-) and sulphates (SO42-) are examples of anions that
are readily leached.
A soil with a low cation exchange capacity can still
be productive through the addition of fertilisers. Nutrients added to a
soil with a low CEC are more likely to be leached quickly.
Figure 4:
Expected
Cation Exchange Capacity of Some Soils - 0-10cm
|
Typical
Soil
|
Expected
CEC
|
Heavy cracking
clays
|
> 20 meq/100g
|
Red brown earths
|
8 - 12 meq/100g
|
Sandy Mallee
|
5 meq/100g
|
Source: Hi-Fert,
Plant Nutrition and Soil Fertility, 1997
|
The combination of cations that make up the exchange
complex is important, with some cations being more desirable than others.
Calcium and potassium are important for soil structure. Too much sodium
indicates a sodicity problem; too much aluminium in low pH soils will
prevent healthy plant growth, eg canola and lucerne.
Figure 5::
Desirable
ranges of cations making up the cation exchange capacity
|
Cation |
Desired Range |
Calcium |
65 - 80% |
Magnesium |
10 - 15% |
Potassium |
1 - 5% |
Sodium |
0 - 4% |
Aluminium |
0 - 1% |
Source: Hi-Fert,
Plant Nutrition and Soil Fertility, 1997
|
3.10 Storage of Water and Nutrients
[ Back ] [ Next ]
|